The CFD simulation showed that the proposed solution would actually not solve the problem, due to some peculiarities in the plant layout. The supplier's engineers used the simulation to evaluate alternatives and came up with a new approach that used different equipment in different locations to overcome the layout issues. They then used simulation to optimize the equipment capacity, providing the required cooling at the lowest possible cost. The solution worked so well that the plastics manufacturer has already installed it at four similar plants located around the world.
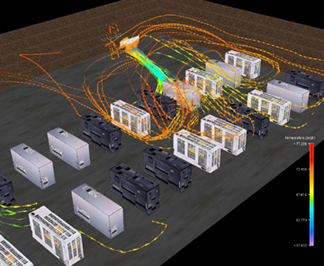
NEED TO PROTECT MACHINERY AND EQUIPMENT
The plastics manufacturer is in the process of building a series of very similar factories. Its manufacturing process uses 52 injection molding machines arranged in two rows of 26 machines each. The computer controls on the machines must be maintained at relatively cool temperatures in order to avoid damage to delicate semiconductors. The materials used in the molding process also have relatively strict temperature requirements.When the company built its first plant, temperatures were far too high for the machines and materials, not to mention the people working in the plant. The company called in a representative from Applied Air, Dallas, to discuss adding cooling equipment to the plant. A limiting factor was that the air space above the machines is utilized by cranes and other equipment, making it impossible to install overhead fans. Also, plant personnel work along the walls that run parallel to the rows of machines, limiting the placement of equipment in that area.
Using hand calculations, the representative proposed a solution that involved putting two Applied Air IFA 60,000 cfm air turnover systems at either end of the building, blowing air from both ends down the length of the machine rows towards the middle. The air supplies were located using extensions 22 feet above the floor and the returns were at floor level. Air turnover systems are designed to address the HVAC challenges of buildings with high ceilings. In these buildings, thermal barriers are established as warm air rises and gets trapped at the ceiling. The temperature at the ceiling may be 25 degrees hotter than at the floor, and the heat trapped at the higher level is unusable. Since the desired building temperature must be maintained at a low level, the inevitable result is excessive energy bills.
Air stratification also creates problems in spaces that require cooling. Because cool air settles to the floor, higher levels remain too warm. When the temperature is not uniform from floor to ceiling, condensation can form, causing moisture damage. An air turnover system solves the air stratification problem by continuously circulating the air in the space. It picks up air at the floor, removing the coldest air layer supporting the thermal barrier. The air is heated or cooled, and returned quietly through a screened discharge plenum to create a uniform temperature and a comfortable space.
SIMULATION HELPS IDENTIFY AND FIX HVAC PROBLEMS
However, because of the special challenges involved in this application, Applied Air recommended to the manufacturer that computer simulation be performed to evaluate the effectiveness of the proposed solution. CFD can calculate and graphically illustrate complete airflow patterns, including velocities and distributions of variables such as pressure and temperature. As part of the analysis, the user may change the layout of the building or the operating conditions, and observe the effect of the changes on the airflow patterns and temperature distribution, and how they impact the cooling performance. Engineers are able to quickly evaluate the performance of alternative equipment configurations.Applied Air has successfully used CFD for three years to analyze HVAC problems and evaluate and optimize potential solutions. The company uses the Flovent software from Flomerics because it is designed specifically for the building industry. "It's much easier modeling building problems with Flovent than a general-purpose CFD code," said Mike Kaler, application engineering manager for Applied Air. "Flovent's technical support staff specializes in HVAC so they can quickly and easily understand what we are trying to do and provide answers."

MODELING THE BUILDING AND EQUIPMENT
The manufacturer supplied a 3D drawing of the building, a floor plan that showed where the equipment was located, and cut sheets that provided specifications of the equipment. Applied Air engineers began by constructing a box representing the building. They then created cubes in the approximate shape of the molding machines and located them in the appropriate positions. To define the amount of heat generated by each machine, they started with its power consumption as provided by the cut sheets. Knowing that the construction of the machine, and particularly how well it is insulated, can have a big effect on the amount of heat actually released, they also interviewed the plant manager. The plant manager said that the surface temperatures of the machines ranged from 140-200°F.Applied Air engineers entered the surface temperature and power consumption into the software, which then calculated the effective heat dissipation. The most important specifications needed to model the air turnover systems were the supply velocity and temperature as well as the physical locations of the supplies and returns. The ambient air temperature outside the plant and insulating properties of the building were also entered.
Applied Air engineers ran a steady-state solution on a personal computer. Engineers viewed the results by looking at temperature mapped as color contour charts across horizontal sections at various levels above the floor. They also took advantage of the ability to set monitoring points in Flovent to capture temperatures in areas that were expected to be difficult to cool. The simulation results showed that the temperatures were still far too high, above 90°F in many areas and above 100°F in a few areas. With traditional hand calculations showing that the proposed equipment should easily cool the space, engineers were curious as to why this configuration didn't work.
DIAGNOSING THE PROBLEM
So they viewed the simulation results as particle traces that are often useful in diagnosing HVAC problems. In this case, the particle traces showed that the hot machines were generating strong convective flow straight up to the ceiling. The horizontal flow generated by the air turnover systems moved directly into this updraft. The horizontal flow from each direction was able to penetrate less than halfway across the length of the machine rows before it was absorbed by the updraft. The result was that the machines in the middle of the building received little or no cooling.With no room for an overhead fan, engineers evaluated the possibility of increasing the capacity of the air turnover units. They set up another simulation run that varied the flow capacity of the turnover units until the temperatures at the monitoring points had reached acceptable levels. This optimization run showed that the cooling units had to be increased to a range of between 90,000 and 120,000 cfm in order to achieve marginally acceptable temperatures. Units of this capacity were not available in the style required to meet the geometrical restrictions of the plant and would have been expensive if they were. So Applied Air engineers looked for another solution.
ITERATING TO AN OPTIMIZED SOLUTION
Based on the diagnostic information that showed the updraft was preventing horizontal flow across the length of the rows, they decided to try a different approach where air was passed across the width of the rows, so it only had to traverse two machines rather than 26. This required switching to DFM indoor units which can be configured for heating, heating and cooling, or just cooling as in this case. They can be mounted on walls well above the floor. This was necessary because the floor along these walls of the plant is devoted to office space. In the simulation, engineers mounted the DFM units about 10 feet from the nearest wall by suspending them from the roof structure to discharge at the 26-foot level. The simulation showed that the DFM units were easily able to penetrate the width of the machine rows and the convective updraft was actually beneficial in this configuration because it prevented the cool air layer from dropping too much. The initial simulation run showed temperatures were at acceptable levels.Not satisfied, Applied Air engineers re-ran the simulation in optimization mode to determine the minimum configuration that would provide acceptable cooling with a margin of safety. This simulation run showed that 60,000 cfm units provided effective cooling at a very attractive cost. The manufacturer installed the cooling units in the plant and was so happy with their performance that it has standardized on them and is using them for each of its plants during its current expansion drive.
For more information, visit Flomerics' Website at www.flovent.com.
Publication date: 06/05/2006
Report Abusive Comment